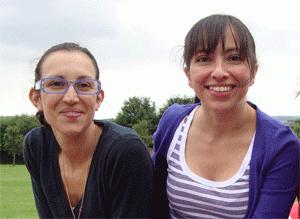
Beatrice and Paola discover that MO25 scaffolding protein has roles beyond regulating the LKB1 tumour suppressor
Beatrice Filippi and Paola de los Heros MRC Unit postdocs working in Dario Alessi's lab have reevaluated the role of the MO25 scaffolding protein, that Jerome Boudeau - a previous postdoc now running a lab in Montpellier - discovered was a key component required for the activation of the LKB1 tumour suppressor complex [1].
MO25 is required to activate LKB1 and is required for LKB1 to phosphorylate and activate its 14 downstream AMPK family kinase substrates [2-4]. MO25 activates LKB1 through its ability to bind to a catalytically inactive pseudokinase termed STRAD, which is related to STE20 family protein kinases [1, 6, 7]. Elton Zeqiraj, a former PhD student in the MRC Unit (now a Sir Henry Wellcome Fellow working in Toronto), showed that MO25 stabilises STRAD in an active conformation that is capable of binding to and stimulating LKB1.
By analyzing in depth the three-dimensional structure and the interface of MO25-STRAD complex, Beatrice realized that other kinases related to STE20 family of protein kinases posses significant homology with the regions of STRAD that interact with MO25. Beatrice focused on studying five of these STRAD related kinases that play vital biological roles in regulating ion homeostasis and blood pressure (SPAK/OSR1) and cell growth and morphogenesis (MST3/MST4/YSK1).
Beatrice was able to demonstrate that five kinases do indeed interact with MO25 isoforms. Working with Paola they showed that remarkably, MO25 binding stimulated the activity of SPAK and OSR1 over 100-fold. In the case of MST3/MST4/YSK1, binding of MO25 increased the activity of these enzymes ~4-fold.
Beatrice showed that MO25 binds to these STRAD-related STE20 kinases with micromolar affinity, and that mutations that prevent the interaction of MO25 with STRAD also impaired binding of MO25 to SPAK/OSR1 and MST3/MST4/YSK1, as well as activation of these enzymes.
Paola was able to demonstrate that ~70% reduction of MO25Ã_± using an siRNA approach in 293 cells inhibited phosphorylation of NKCC1, a physiological substrate of SPAK/OSR1 kinases. Importantly this inhibitory effect was rescued following re-expression of MO25.
Paola also observed that SPAK/OSR1 phosphorylates the three identified substrates of this enzyme (NKCC, NKCC2 and NCC) at a vastly higher rate in the presence of MO25. Significantly, phosphorylation site analysis led to the identification of novel in vitro phosphorylation sites not observed in previous phosphorylation studies undertaken in the absence of MO25. Most of the new reported phosphorylation sites such as Ser71 (mouse sequence of NCC, Ser73 in human sequence) [8] have been identified to be phosphorylated in vivo, following stimulation of cells or tissues with osmotic shock conditions that activate the SPAK/OSR1 pathway. Paola's new data therefore suggests that SPAK/OSR1 are likely to represent the physiological kinases that phosphorylate these residues rather than another kinase.
There is also great interest in generating inhibitors of SPAK/OSR1 for the treatment of hypertension. The finding that these enzymes can be activated 100-fold by binding to MO25 will be vital for enabling these inhibitor studies. We suggest that screening for SPAK/OSR1 inhibitors are undertaken with the SPAK-MO25 or OSR1-MO25 complex rather than isolated SPAK or OSR1.
Recent findings by Sean Lawler research group at Columbus Ohio (who incidentally was a previous MRC Unit postdoc working with Philip Cohen) revealed that a microRNA (miR-451) that promoted growth of gliomas by inhibiting expression of the MO25Ã_± isoform [5]. The effects of miR-451 on cancer cells were interpreted to be all mediated through inhibition of the LKB1 tumour suppressor. However, our new data indicate that the effects of miR-451 are also likely to be contributed to the inhibition of other STE20 kinases that are regulated by MO25.
Our data suggests that MO25 evolved as an ancestral regulator of the active conformation of kinases as well as pseudokinases. It is likely that MO25 may interact with additional kinases that we have not investigated in our study. We also discuss a controversial idea that the original kinase may have evolved from a pseudokinase whose role could have
been to interact with targets in a manner regulated by MO25 and ATP binding.
To read a copy of Beatrice's and Paola's paper click here.
Beatrice has recently started working as a postdoc in the MaRS Centre in Toronto.
References
1 Boudeau, J., Baas, A. F., Deak, M., Morrice, N. A., Kieloch, A., Schutkowski, M., Prescott, A. R., Clevers, H. C. and Alessi, D. R. (2003) MO25alpha/beta interact with STRADalpha/beta enhancing their ability to bind, activate and localize LKB1 in the cytoplasm. EMBO J. 22, 5102-5114
2 Hawley, S. A., Boudeau, J., Reid, J. L., Mustard, K. J., Udd, L., Makela, T. P., Alessi, D. R. and Hardie, D. G. (2003) Complexes between the LKB1 tumor suppressor, STRADalpha/beta and MO25alpha/beta are upstream kinases in the AMP-activated protein kinase cascade. J Biol. 2, 28
3 Jaleel, M., McBride, A., Lizcano, J. M., Deak, M., Toth, R., Morrice, N. A. and Alessi, D. R. (2005) Identification of the sucrose non-fermenting related kinase SNRK, as a novel LKB1 substrate. FEBS Lett. 579, 1417-1423
4 Lizcano, J. M., Goransson, O., Toth, R., Deak, M., Morrice, N. A., Boudeau, J., Hawley, S. A., Udd, L., Makela, T. P., Hardie, D. G. and Alessi, D. R. (2004) LKB1 is a master kinase that activates 13 kinases of the AMPK subfamily, including MARK/PAR-1. EMBO J. 23, 833-843
5 Godlewski, J., Nowicki, M. O., Bronisz, A., Nuovo, G., Palatini, J., De Lay, M., Van Brocklyn, J., Ostrowski, M. C., Chiocca, E. A. and Lawler, S. E. (2010) MicroRNA-451 regulates LKB1/AMPK signaling and allows adaptation to metabolic stress in glioma cells. Mol Cell. 37, 620-632
6 Boudeau, J., Miranda-Saavedra, D., Barton, G. J. and Alessi, D. R. (2006) Emerging roles of pseudokinases. Trends Cell Biol. 16, 443-452
7 Boudeau, J., Scott, J. W., Resta, N., Deak, M., Kieloch, A., Komander, D., Hardie, D. G., Prescott, A. R., van Aalten, D. M. and Alessi, D. R. (2004) Analysis of the LKB1-STRAD-MO25 complex. J Cell Sci. 117, 6365-6375
8 Yang, S. S., Morimoto, T., Rai, T., Chiga, M., Sohara, E., Ohno, M., Uchida, K., Lin, S. H., Moriguchi, T., Shibuya, H., Kondo, Y., Sasaki, S. and Uchida, S. (2007) Molecular pathogenesis of pseudohypoaldosteronism type II: generation and analysis of a Wnk4(D561A/+) knockin mouse model. Cell Metab. 5, 331-344
MO25 is required to activate LKB1 and is required for LKB1 to phosphorylate and activate its 14 downstream AMPK family kinase substrates [2-4]. MO25 activates LKB1 through its ability to bind to a catalytically inactive pseudokinase termed STRAD, which is related to STE20 family protein kinases [1, 6, 7]. Elton Zeqiraj, a former PhD student in the MRC Unit (now a Sir Henry Wellcome Fellow working in Toronto), showed that MO25 stabilises STRAD in an active conformation that is capable of binding to and stimulating LKB1.
By analyzing in depth the three-dimensional structure and the interface of MO25-STRAD complex, Beatrice realized that other kinases related to STE20 family of protein kinases posses significant homology with the regions of STRAD that interact with MO25. Beatrice focused on studying five of these STRAD related kinases that play vital biological roles in regulating ion homeostasis and blood pressure (SPAK/OSR1) and cell growth and morphogenesis (MST3/MST4/YSK1).
Beatrice was able to demonstrate that five kinases do indeed interact with MO25 isoforms. Working with Paola they showed that remarkably, MO25 binding stimulated the activity of SPAK and OSR1 over 100-fold. In the case of MST3/MST4/YSK1, binding of MO25 increased the activity of these enzymes ~4-fold.
Beatrice showed that MO25 binds to these STRAD-related STE20 kinases with micromolar affinity, and that mutations that prevent the interaction of MO25 with STRAD also impaired binding of MO25 to SPAK/OSR1 and MST3/MST4/YSK1, as well as activation of these enzymes.
Paola was able to demonstrate that ~70% reduction of MO25Ã_± using an siRNA approach in 293 cells inhibited phosphorylation of NKCC1, a physiological substrate of SPAK/OSR1 kinases. Importantly this inhibitory effect was rescued following re-expression of MO25.
Paola also observed that SPAK/OSR1 phosphorylates the three identified substrates of this enzyme (NKCC, NKCC2 and NCC) at a vastly higher rate in the presence of MO25. Significantly, phosphorylation site analysis led to the identification of novel in vitro phosphorylation sites not observed in previous phosphorylation studies undertaken in the absence of MO25. Most of the new reported phosphorylation sites such as Ser71 (mouse sequence of NCC, Ser73 in human sequence) [8] have been identified to be phosphorylated in vivo, following stimulation of cells or tissues with osmotic shock conditions that activate the SPAK/OSR1 pathway. Paola's new data therefore suggests that SPAK/OSR1 are likely to represent the physiological kinases that phosphorylate these residues rather than another kinase.
There is also great interest in generating inhibitors of SPAK/OSR1 for the treatment of hypertension. The finding that these enzymes can be activated 100-fold by binding to MO25 will be vital for enabling these inhibitor studies. We suggest that screening for SPAK/OSR1 inhibitors are undertaken with the SPAK-MO25 or OSR1-MO25 complex rather than isolated SPAK or OSR1.
Recent findings by Sean Lawler research group at Columbus Ohio (who incidentally was a previous MRC Unit postdoc working with Philip Cohen) revealed that a microRNA (miR-451) that promoted growth of gliomas by inhibiting expression of the MO25Ã_± isoform [5]. The effects of miR-451 on cancer cells were interpreted to be all mediated through inhibition of the LKB1 tumour suppressor. However, our new data indicate that the effects of miR-451 are also likely to be contributed to the inhibition of other STE20 kinases that are regulated by MO25.
Our data suggests that MO25 evolved as an ancestral regulator of the active conformation of kinases as well as pseudokinases. It is likely that MO25 may interact with additional kinases that we have not investigated in our study. We also discuss a controversial idea that the original kinase may have evolved from a pseudokinase whose role could have
been to interact with targets in a manner regulated by MO25 and ATP binding.
To read a copy of Beatrice's and Paola's paper click here.
Beatrice has recently started working as a postdoc in the MaRS Centre in Toronto.
References
1 Boudeau, J., Baas, A. F., Deak, M., Morrice, N. A., Kieloch, A., Schutkowski, M., Prescott, A. R., Clevers, H. C. and Alessi, D. R. (2003) MO25alpha/beta interact with STRADalpha/beta enhancing their ability to bind, activate and localize LKB1 in the cytoplasm. EMBO J. 22, 5102-5114
2 Hawley, S. A., Boudeau, J., Reid, J. L., Mustard, K. J., Udd, L., Makela, T. P., Alessi, D. R. and Hardie, D. G. (2003) Complexes between the LKB1 tumor suppressor, STRADalpha/beta and MO25alpha/beta are upstream kinases in the AMP-activated protein kinase cascade. J Biol. 2, 28
3 Jaleel, M., McBride, A., Lizcano, J. M., Deak, M., Toth, R., Morrice, N. A. and Alessi, D. R. (2005) Identification of the sucrose non-fermenting related kinase SNRK, as a novel LKB1 substrate. FEBS Lett. 579, 1417-1423
4 Lizcano, J. M., Goransson, O., Toth, R., Deak, M., Morrice, N. A., Boudeau, J., Hawley, S. A., Udd, L., Makela, T. P., Hardie, D. G. and Alessi, D. R. (2004) LKB1 is a master kinase that activates 13 kinases of the AMPK subfamily, including MARK/PAR-1. EMBO J. 23, 833-843
5 Godlewski, J., Nowicki, M. O., Bronisz, A., Nuovo, G., Palatini, J., De Lay, M., Van Brocklyn, J., Ostrowski, M. C., Chiocca, E. A. and Lawler, S. E. (2010) MicroRNA-451 regulates LKB1/AMPK signaling and allows adaptation to metabolic stress in glioma cells. Mol Cell. 37, 620-632
6 Boudeau, J., Miranda-Saavedra, D., Barton, G. J. and Alessi, D. R. (2006) Emerging roles of pseudokinases. Trends Cell Biol. 16, 443-452
7 Boudeau, J., Scott, J. W., Resta, N., Deak, M., Kieloch, A., Komander, D., Hardie, D. G., Prescott, A. R., van Aalten, D. M. and Alessi, D. R. (2004) Analysis of the LKB1-STRAD-MO25 complex. J Cell Sci. 117, 6365-6375
8 Yang, S. S., Morimoto, T., Rai, T., Chiga, M., Sohara, E., Ohno, M., Uchida, K., Lin, S. H., Moriguchi, T., Shibuya, H., Kondo, Y., Sasaki, S. and Uchida, S. (2007) Molecular pathogenesis of pseudohypoaldosteronism type II: generation and analysis of a Wnk4(D561A/+) knockin mouse model. Cell Metab. 5, 331-344